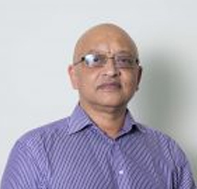
Name: Prim Singh
Email: prim.singh@nu.edu.kz
Department of Biomedical Sciences
CV and research interest
Obtained PhD in 1986 from Cambridge University and have headed groups in Cambridge (UK), Edinburgh (UK), Hamburg (Germany), Berlin (Germany), Novosibirsk (Russia) and Nur-Sultan (Kazakhstan). Raised ~$8M in funding from Academia, Industry and Charities. I have two areas of research interest:
- Polymer-polymer phase separation and synthetic biology
We have proposed a new paradigm for understanding how compartmentalization of the genome into heterochromatin and euchromatin is directed by polymer-polymer phase separation mediated by HP1 proteins (publication 1 below; submitted). Our approach is based on polymer physics, where HP1-containing heterochromatin domains/complexes are treated as “blocks” in a block co-polymers (BCPs). Notably, work on synthetic BCPs has attracted considerable attention because they can self-assemble into ordered nanostructures that have considerable practical utility. The stage is therefore set for a new synthetic biology where manipulation of HP1 proteins can be used to drive assembly of different nanostructures that can regulate the 3D organisation of the genome.
- Age reprogramming and epigenetic rejuvenation
Age reprogramming represents a novel method for generating patient-specific tissues for transplantation – see publications 2 and 4 below. Age reprogramming bypasses the de-differentiation/re-differentiation cycle that is characteristic of the induced pluripotent stem (iPS) and nuclear transfer-embryonic stem (NT-ES) cell technologies that drive current interest in regenerative medicine. Instead, age reprogramming aims to rejuvenate the specialized functions of an old cell without de-differentiation; age reprogramming does not require developmental reprogramming through an embryonic stage, unlike the iPS and NT-ES cell-based therapies.
Project(s) for intake 2021:
- Elucidation of the role of Lamin-associated proteins in cellular senescence using CRISPR-Cas9 technology
Overview:
Cellular senescence is an irreversible cell-cycle arrest, originally described for primary cells after long-term cell culture [1]. A role for senescence in organismal aging has recently been substantiated; the depletion of senescent cells has been shown to relieve symptoms in mouse models of age-related diseases, suggesting that cellular senescence may be a useful model system for organismal aging [2,3]. Cellular senescence in normal human diploid fibroblasts (HDFs) is accompanied by a large-scale spatial rearrangement of chromatin, forming nuclear structures known as senescence-associated heterochromatic foci (SAHF). SAHF are enriched in constitutive heterochromatic markers, such as H3K9me3 and HP1 proteins [4]. SAHF formation is also associated with dramatic depletion of lamin B1 [5,6] and the lamin B receptor [7].
To date, studies of the effect of depletion of HP1 proteins, histone methyltrasferases (HMTases) that generate H3K9me3, and the membrane associated proteins Lamin B and Lamin B receptor (LBR) have not been undertaken in primary HDFs, which are the “gold-standard” for in vitro study of cellular senescence [8]. Here, we will specifically disrupt the genes encoding the Lamin B1 and LBR in HDFs and test the effect on cellular senescence.
Accordingly, the aim of this project is to elucidate, in concrete molecular and cellular terms, the role of lamin-associated proteins in cellular senescence. Specifically, our aims are to:
- Disrupt Lamin B1 and LBR genes individually and together in HDFs using the CRISPR-Cas9 system.
- Test the effect of single and compound lamin mutants on: (a) replicative senescence; (b) histone modifications; (c) DNA methylation; (d) Hi-C maps.
Potential impact:
We will have determined the role played by lamin-associated proteins in cellular senescence and this is likely to focus attention to current rejuvenation technologies. This could enhance age reprogramming efficiency with all the attendant benefits for Medicine in general and regenerative therapies in particular.
Techniques:
The CRISPR-Cas9 constructs will be made according to standard protocols (see [9]); the laboratory has extensive experience in gene targeting [10,11]. Characterization of the lamin gene disruption(s) will include measurement of lifespan by population doublings and telomere length [12]. Measurement of epigenetic modifications (ChIP-Seq and DNA methylation analysis) and genome organization (Hi-C) will also be undertaken to fully characterize the mutations.
References:
- Hayflick, L., and Moorhead, P.S. (1961). The serial cultivation of human diploid cell strains. Exp. Cell Res. 25, 585–621.
- Baker, D.J., Wijshake, T., Tchkonia, T., LeBrasseur, N.K., Childs, B.G., van de Sluis, B., Kirkland, J.L., and van Deursen, J.M. (2011). Clearance of p16Ink4apositive senescent cells delays ageing-associated disorders. Nature 479, 232–236.
- Lopez-Otın, C., Blasco, M.A., Partridge, L., Serrano, M., and Kroemer, G. (2013). The hallmarks of aging. Cell 153, 1194–1217.
- Narita, M., Nunez, S., Heard, E., Narita, M., Lin, A.W., Hearn, S.A., Spector, D.L., Hannon, G.J., and Lowe, S.W. (2003). Rb-mediated heterochromatin formation and silencing of E2F target genes during cellular senescence. Cell 113, 703–716
- Sadaie, M., Salama, R., Carroll, T., Tomimatsu, K., Chandra, T., Young, A.R.J., Narita, M., Pe´ rez-Mancera, P.A., Bennett, D.C., Chong, H., et al. (2013). Redistribution of the Lamin B1 genomic binding profile affects rearrangement of heterochromatic domains and SAHF formation during senescence. Genes Dev. 27, 1800–1808.
- Shah, P.P., Donahue, G., Otte, G.L., Capell, B.C., Nelson, D.M., Cao, K., Aggarwala, V., Cruickshanks, H.A., Rai, T.S., McBryan, T., et al. (2013). Lamin B1 depletion in senescent cells triggers large-scale changes in gene expression and the chromatin landscape. Genes Dev. 27, 1787–1799.
- Lukášová E., Kovarík, A. and Stanislav Kozubek S. (2018). Consequences of Lamin B1 and Lamin B Receptor Downregulation in Senescence. Cells 7, 11-20.
- Sikora, E., Arendt, T., Bennett, M. and Narita, M. (2011). Impact of cellular senescence signature on ageing research. Ageing Res. Rev. 10, 146–52.
- RanFA, Hsu PD, Wright J, Agarwala V, Scott DA and Zhang F. (2013). Genome engineering using the CRISPR-Cas9 system. Nature Protocols, 8, 2281-2308.
- Aucott R., Bullwinkel J., Yu Y., Shi W., Billur M., Brown J.P., Menzel U., Kioussis D., Wang G., Reisert I., Weimer J., Pandita R.K., Sharma G.G., Pandita T.K., Fundele R. and Singh (2008). HP1-beta is required for development of the cerebral neocortex and neuromuscular junctions. 183(4), 597-606.
- Brown JP, Bullwinkel J, Baron-Lühr B, Billur M, Schneider P, Winking H, Singh (2010). HP1gamma function is required for male germ cell survival and spermatogenesis. Epigenetics and Chromatin. 3(1), 9.
- Brown JP, Wei W, Sedivy (1997). Bypass of senescence after disruption of p21CIP1/WAF1 gene in normal diploid human fibroblasts. Science. 277(5327), 831-4.
- Elucidation of the role of histone methyltransferase (HMTase) proteins in cellular senescence using CRISPR-Cas9 technology
Overview:
Cellular senescence is an irreversible cell-cycle arrest, originally described for primary cells after long-term cell culture [1]. A role for senescence in organismal aging has recently been substantiated; the depletion of senescent cells has been shown to relieve symptoms in mouse models of age-related diseases, suggesting that cellular senescence may be a useful model system for organismal aging [2,3]. Cellular senescence in normal human diploid fibroblasts (HDFs) is accompanied by a large-scale spatial rearrangement of chromatin, forming nuclear structures known as senescence-associated heterochromatic foci (SAHF). SAHF are enriched in constitutive heterochromatic markers, such as H3K9me3 and HP1 proteins [4]. SAHF formation is also associated with dramatic depletion of lamin B1 [5,6] and the lamin B receptor [7].
To date, studies of the effect of depletion of HP1 proteins, histone methyltrasferases (HMTases) that generate H3K9me3, and the membrane associated proteins Lamin B and Lamin B receptor (LBR) have not been undertaken in primary HDFs, which are the “gold-standard” for in vitro study of cellular senescence [8]. Here, we will specifically disrupt the genes encoding the HMTase proteins - Suv(3)9h1/2, SETDB1, G9a and GLP – in HDFs and test the effect on cellular senescence.
Accordingly, the aim of this project is to elucidate, in concrete molecular and cellular terms, the role of HMTase proteins in cellular senescence. Specifically, our aims are to:
- Disrupt Suv(3)9h1/2, SETDB1, G9a and GLP genes individually and collectively in HDFs using the CRISPR-Cas9 system
- Test the effect of single and compound HMTase mutants on: (a) replicative senescence; (b) histone modifications; (c) DNA methylation; (d) Hi-C maps.
Potential impact:
We will have determined the role played by HMTase proteins in cellular senescence and this is likely to focus attention to current rejuvenation technologies. This could enhance age reprogramming efficiency with all the attendant benefits for Medicine in general and regenerative therapies in particular.
Techniques:
The CRISPR-Cas9 constructs will be made according to standard protocols (see [9]); the laboratory has extensive experience in gene targeting [10,11]. Characterization of the HMTase gene disruption(s) will include measurement of lifespan by population doublings and telomere length [12]. Measurement of epigenetic modifications (ChIP-Seq and DNA methylation analysis) and genome organization (Hi-C) will also be undertaken to fully characterize the mutations.
References:
- Hayflick, L., and Moorhead, P.S. (1961). The serial cultivation of human diploid cell strains. Exp. Cell Res. 25, 585–621.
- Baker, D.J., Wijshake, T., Tchkonia, T., LeBrasseur, N.K., Childs, B.G., van de Sluis, B., Kirkland, J.L., and van Deursen, J.M. (2011). Clearance of p16Ink4apositive senescent cells delays ageing-associated disorders. Nature 479, 232–236.
- Lopez-Otın, C., Blasco, M.A., Partridge, L., Serrano, M., and Kroemer, G. (2013). The hallmarks of aging. Cell 153, 1194–1217.
- Narita, M., Nunez, S., Heard, E., Narita, M., Lin, A.W., Hearn, S.A., Spector, D.L., Hannon, G.J., and Lowe, S.W. (2003). Rb-mediated heterochromatin formation and silencing of E2F target genes during cellular senescence. Cell 113, 703–716
- Sadaie, M., Salama, R., Carroll, T., Tomimatsu, K., Chandra, T., Young, A.R.J., Narita, M., Pe´ rez-Mancera, P.A., Bennett, D.C., Chong, H., et al. (2013). Redistribution of the Lamin B1 genomic binding profile affects rearrangement of heterochromatic domains and SAHF formation during senescence. Genes Dev. 27, 1800–1808.
- Shah, P.P., Donahue, G., Otte, G.L., Capell, B.C., Nelson, D.M., Cao, K., Aggarwala, V., Cruickshanks, H.A., Rai, T.S., McBryan, T., et al. (2013). Lamin B1 depletion in senescent cells triggers large-scale changes in gene expression and the chromatin landscape. Genes Dev. 27, 1787–1799.
- Lukášová E., Kovarík, A. and Stanislav Kozubek S. (2018). Consequences of Lamin B1 and Lamin B Receptor Downregulation in Senescence. Cells 7, 11-20.
- Sikora, E., Arendt, T., Bennett, M. and Narita, M. (2011). Impact of cellular senescence signature on ageing research. Ageing Res. Rev. 10, 146–52.
- RanFA, Hsu PD, Wright J, Agarwala V, Scott DA and Zhang F. (2013). Genome engineering using the CRISPR-Cas9 system. Nature Protocols, 8, 2281-2308.
- Aucott R., Bullwinkel J., Yu Y., Shi W., Billur M., Brown J.P., Menzel U., Kioussis D., Wang G., Reisert I., Weimer J., Pandita R.K., Sharma G.G., Pandita T.K., Fundele R. and Singh (2008). HP1-beta is required for development of the cerebral neocortex and neuromuscular junctions. 183(4), 597-606.
- Brown JP, Bullwinkel J, Baron-Lühr B, Billur M, Schneider P, Winking H, Singh (2010). HP1gamma function is required for male germ cell survival and spermatogenesis. Epigenetics and Chromatin. 3(1), 9.
- Brown JP, Wei W, Sedivy (1997). Bypass of senescence after disruption of p21CIP1/WAF1 gene in normal diploid human fibroblasts. Science. 277(5327), 831-4.
- Elucidation of the role of HP1 proteins in cellular senescence using CRISPR-Cas9 technology
Overview:
Cellular senescence is an irreversible cell-cycle arrest, originally described for primary cells after long-term cell culture [1]. A role for senescence in organismal aging has recently been substantiated; the depletion of senescent cells has been shown to relieve symptoms in mouse models of age-related diseases, suggesting that cellular senescence may be a useful model system for organismal aging [2,3]. Cellular senescence in normal human diploid fibroblasts (HDFs) is accompanied by a large-scale spatial rearrangement of chromatin, forming nuclear structures known as senescence-associated heterochromatic foci (SAHF). SAHF are enriched in constitutive heterochromatic markers, such as H3K9me3 and HP1 proteins [4]. SAHF formation is also associated with dramatic depletion of lamin B1 [5,6] and the lamin B receptor [7].
To date, studies of the effect of depletion of HP1 proteins, histone methyltrasferases that generate H3K9me3, and the membrane associated proteins Lamin B and Lamin B receptor (LBR) have not been undertaken in primary HDFs, which are the “gold-standard” for in vitro study of cellular senescence [8]. Here, we will specifically disrupt the genes encoding the HP1 proteins in HDFs and test the effect on cellular senescence, epigenetic modifications and genome organization.
Accordingly, the aim of this project is to elucidate, in concrete molecular and cellular terms, the role of HP1 proteins in cellular senescence. Specifically, our aims are to:
- Disrupt HP1 genes (a, b and g) individually and collectively in HDFs using the CRISPR-Cas9 system
- Test the effect of single and compound HP1 mutants on: (a) replicative senescence; (b) histone modifications; (c) DNA methylation; (d) Hi-C maps.
Potential impact:
We will have determined the role played by HP1 proteins in cellular senescence and this is likely to focus attention to current rejuvenation technologies. This could enhance age reprogramming efficiency with all the attendant benefits for Medicine in general and regenerative therapies in particular.
Techniques:
The CRISPR-Cas9 constructs will be made according to standard protocols (see [9]); the laboratory has extensive experience in gene targeting ([10,11]). Characterization of the HP1 gene disruption(s) will include measurement of lifespan by population doublings and telomere length [12]. Measurement of epigenetic modifications (ChIP-Seq and DNA methylation analysis) and genome organization (Hi-C) will also be undertaken to fully characterize the mutations.
References:
- Hayflick, L., and Moorhead, P.S. (1961). The serial cultivation of human diploid cell strains. Exp. Cell Res. 25, 585–621.
- Baker, D.J., Wijshake, T., Tchkonia, T., LeBrasseur, N.K., Childs, B.G., van de Sluis, B., Kirkland, J.L., and van Deursen, J.M. (2011). Clearance of p16Ink4apositive senescent cells delays ageing-associated disorders. Nature 479, 232–236.
- Lopez-Otın, C., Blasco, M.A., Partridge, L., Serrano, M., and Kroemer, G. (2013). The hallmarks of aging. Cell 153, 1194–1217.
- Narita, M., Nunez, S., Heard, E., Narita, M., Lin, A.W., Hearn, S.A., Spector, D.L., Hannon, G.J., and Lowe, S.W. (2003). Rb-mediated heterochromatin formation and silencing of E2F target genes during cellular senescence. Cell 113, 703–716
- Sadaie, M., Salama, R., Carroll, T., Tomimatsu, K., Chandra, T., Young, A.R.J., Narita, M., Pe´ rez-Mancera, P.A., Bennett, D.C., Chong, H., et al. (2013). Redistribution of the Lamin B1 genomic binding profile affects rearrangement of heterochromatic domains and SAHF formation during senescence. Genes Dev. 27, 1800–1808.
- Shah, P.P., Donahue, G., Otte, G.L., Capell, B.C., Nelson, D.M., Cao, K., Aggarwala, V., Cruickshanks, H.A., Rai, T.S., McBryan, T., et al. (2013). Lamin B1 depletion in senescent cells triggers large-scale changes in gene expression and the chromatin landscape. Genes Dev. 27, 1787–1799.
- Lukášová E., Kovarík, A. and Stanislav Kozubek S. (2018). Consequences of Lamin B1 and Lamin B Receptor Downregulation in Senescence. Cells 7, 11-20.
- Sikora, E., Arendt, T., Bennett, M. and Narita, M. (2011). Impact of cellular senescence signature on ageing research. Ageing Res. Rev. 10, 146–52.
- RanFA, Hsu PD, Wright J, Agarwala V, Scott DA and Zhang F. (2013). Genome engineering using the CRISPR-Cas9 system. Nature Protocols, 8, 2281-2308.
- Aucott R., Bullwinkel J., Yu Y., Shi W., Billur M., Brown J.P., Menzel U., Kioussis D., Wang G., Reisert I., Weimer J., Pandita R.K., Sharma G.G., Pandita T.K., Fundele R. and Singh (2008). HP1-beta is required for development of the cerebral neocortex and neuromuscular junctions. 183(4), 597-606.
- Brown JP, Bullwinkel J, Baron-Lühr B, Billur M, Schneider P, Winking H, Singh (2010). HP1gamma function is required for male germ cell survival and spermatogenesis. Epigenetics and Chromatin. 3(1), 9.
- Brown JP, Wei W, Sedivy (1997). Bypass of senescence after disruption of p21CIP1/WAF1 gene in normal diploid human fibroblasts. Science. 277(5327), 831-4.
- Age reprogramming – a scientific approach to cellular rejuvenation 1
Overview:
Age reprogramming enables old cells to be rejuvenated without passage through an embryonic stage [1-3]. As such, age reprogramming stands apart from the induced pluripotent stem (iPS) and nuclear transfer-embryonic stem (NT-ES) cell therapies where histo-compatible cells are produced only after passage through an embryonic stage. In this study we will determine the time course for rejuvenation of five hallmarks of ageing, specifically telomere rejuvenation, mitochondrial rejuvenation, DNA damage reduction, cellular senescence reversal and proteostasis rejuvenation.
There are nine candidate molecular hallmarks of ageing that likely represent evolutionarily-conserved determinants of organismal ageing (see [4]). Each candidate hallmark fulfills, to a lesser or greater extent, the following criteria: ‘(i) it should manifest during normal aging; (ii) its experimental aggravation should accelerate aging; and (iii) its experimental amelioration should retard the normal aging process and, hence, increase healthy lifespan.’ In this PhD proposal we will examine the rejuvenation of five of these hallmarks.
Accordingly, the aim of this project is to elucidate, in concrete molecular and cellular terms, the rejuvenation of telomeres, mitochondrial membrane potential, DNA damage, cellular senescence and proteostasis.
Specifically, our aims are to:
- Determination of the status of telomere length, mitochondrial membrane potential, DNA damage, cellular senescence and proteostasis activity in young and old cells.
- Determine the timing of rejuvenation of telomere length, mitochondrial membrane potential, DNA damage, cellular senescence and proteostasis activity in old cells.
Potential impact:
The ability to routinely make old cells young will have a profound effect on Medicine in general and regenerative therapies in particular [1-3].
Techniques:
Culture of human cells, iPS cell technology, assays for measuring telomere length, mitochondrial membrane potential, DNA damage, cellular senescence and proteostasis.
References:
- Singh, P.B. and Zacouto, F. Nuclear Reprogramming and Epigenetic Rejuvenation. Biosciences 35(2):315-319, 2010.
- Maria Manukyan and Prim B. Singh. Epigenetic rejuvenation. Genes to Cells, 17(5):337-43, 2012.
- Prim B. Singh and Andrew G. Age reprogramming and epigenetic rejuvenation. Epigenetics and Chromatin. 11(1):73, 2018.
- Lopez-Otin C, Blasco MA, Partridge L, Serrano M and Kroemer G. The hallmarks of aging. Cell 153 1194–1217, 2013.
- Age reprogramming – a scientific approach to cellular rejuvenation 2
Overview:
Age reprogramming enables old cells to be rejuvenated without passage through an embryonic stage [1-3]. As such, age reprogramming stands apart from the induced pluripotent stem (iPS) and nuclear transfer-embryonic stem (NT-ES) cell therapies where histo-compatible cells are produced only after passage through an embryonic stage. In this study we will determine the time course for rejuvenation of the epigenome and genome organization.
There are nine candidate molecular hallmarks of ageing that likely represent evolutionarily-conserved determinants of organismal ageing (see [4]). Each candidate hallmark fulfills, to a lesser or greater extent, the following criteria: ‘(i) it should manifest during normal aging; (ii) its experimental aggravation should accelerate aging; and (iii) its experimental amelioration should retard the normal aging process and, hence, increase healthy lifespan.’ In this PhD proposal we will examine the rejuvenation of the epigenome and genome organization.
Accordingly, the aim of this project is to elucidate, in concrete molecular terms, the rejuvenation of epigenome and genome organization..
Specifically, our aims are to:
- Determination of the status of the epigenome (histone modifications; DNA methylation, transcriptome) in young and old cells.
- Determination of genome organization (using Hi-C maps) in young and old cells.
- Determine the timing of rejuvenation the epigenome and genome organization in old cells.
Potential impact:
The ability to routinely make old cells young will have a profound effect on Medicine in general and regenerative therapies in particular [1-3].
Techniques:
Culture of human cells, iPS cell technology, ChIP-Seq, RNA-Seq, DNA methylation micro-array and Hi-C maps.
References:
- Singh, P.B. and Zacouto, F. Nuclear Reprogramming and Epigenetic Rejuvenation. Biosciences 35(2):315-319, 2010.
- Maria Manukyan and Prim B. Singh. Epigenetic rejuvenation. Genes to Cells, 17(5):337-43, 2012.
- Prim B. Singh and Andrew G. Age reprogramming and epigenetic rejuvenation. Epigenetics and Chromatin. 11(1):73, 2018.
- Lopez-Otin C, Blasco MA, Partridge L, Serrano M and Kroemer G. The hallmarks of aging. Cell 153 1194–1217, 2013.
- A molecular basis for B-type heterochromatic compartmental domains in mammalian interphase nuclei.
Overview:
In mammals, two meters of DNA must be folded into the confines of a nucleus that is only five millionths of a meter in diameter. A measure of folding can be assessed by Hi-C, a high-throughput technique that generates contact frequency (Hi-C) maps [1]. Hi-C maps revealed that mammalian DNA is folded into compartmental domains, where A-type compartments represent euchromatin and B-type compartments represent heterochromatin [1]. According to current concepts HP1-containing heterochromatin-like complexes are likely to generate the B-type heterochromatic compartmental domains [2, 3, 4]. The purpose of this proposal is to test this hypothesis. Specifically, our objective is to use HP1 mutant mouse embryonic stem (ES) cells to test the effect of disrupting HP1genes on the B-type heterochromatic compartmental domains observed in Hi-C maps. The proposal is entirely novel. The elucidation of the mechanism that generates the B-type compartmental domains will provide a concrete understanding of how DNA is folded into the confines of mammalian nucleus.
Potential impact:
The knowledge gained from these studies has clear practical importance. The ability to manipulate nuclear organisation lies at the heart of strategies to experimentally change cellular identity particularly within the context of personalized/precision medicine. Understanding how DNA is folded into the nucleus will allow us to determine how it is likely to be misfolded during disease, such as in viral infections (e.g. COVID-19 infection). Accordingly, any advance in this project will have considerable benefits for Medicine.
Techniques:
Culture of (mutant) mouse ES cells;
Generation of Hi-C maps from wt and mutant ES cells;
Bioinformatic analyses
References:
- Dekker, J. and Mirny, LA. The 3D genome as moderator of chromosomal communication. Cell. 2016, 164(6):1110-1121.
- Hildebrand EM and Dekker J, Mechanisms and Functions of ChromosomeCompartmentalization. Trends in Biochemical Sciences. 2020 https://doi.org/10.1016/j.tibs.2020.01.002
- Singh PB, Newman AG. On the relations of phase separation and Hi-C maps to epigenetics. R. Soc. open sci. 2020, 7: 191976. http://dx.doi.org/10.1098/rsos.191976
- 4. Singh PB, Belyakin SN and Laktionov PP. Biology and physics of heterochromatin-like domains/complexes. Cells. 9(8):E1881. 2020. doi: 10.3390/cells9081881